Organic Chemists from Industry and academics to Interact on Spectroscopy Techniques for Organic Compounds ie NMR, MASS, IR, UV Etc. Starters, Learners, advanced, all alike, contains content which is basic or advanced, by Dr Anthony Melvin Crasto, Worlddrugtracker, email me ........... amcrasto@gmail.com, call +91 9323115463 India skype amcrasto64
................DR ANTHONY MELVIN CRASTO Ph.D ( ICT, Mumbai) , INDIA 25Yrs Exp. in the feld of Organic Chemistry,Working for GLENMARK GENERICS at Navi Mumbai, INDIA. Serving chemists around the world. Helping them with websites on Chemistry.Million hits on google, world acclamation from industry, academia, drug authorities for websites, blogs and educational contribution
Pages
- Home
- ABOUT ME
- DIMENSIONS IN NMR SPECTROSCOPY
- 13 C NMR
- 1H NMR
- CHEMDOODLE/INTERACTIVE SPECT PREDICT
- Animations
- HELP ME
- Multinuclear NMR Spectroscopy
- Examples of 13C NMR
- Books on NMR spectroscopy
- UV-Visible Spectroscopy
- IR SPECTRA EXAMPLES
- Journals
- Organic spectroscopy site
- Spectroscopy sites
- IR SPECTROSCOPY
- Books-2
- Recommended Web Sites for Spectra and Spectrum-rel...
- DISCLAIMER
- Mössbauer spectroscopy
- FINDING CHEMICAL SPECTRA
- Mass Spectrometry
- NMR Overview
- Characterisation of Organic Compounds
- SDBS Spectral Database System for Organic Compounds
- CHEMICAL SHIFT
- MASS SPECTROSCOPY
- Books-1
- MASSBANK PORTAL
- 11B NMR
Saturday, 28 October 2017
HR-MAS NMR Spectroscopy in Material Science

The gradient 2D 1 H HR-MAS NMR COSY spectrum for the ionic liquid [MBPyrr] + [TFSI] -

The 1 H HR-MAS NMR of the ionic liquid [MBPyrr] + TFSI - at 298K as a A) neat liquid and B)

Pictorial representation of the gradient produced along the magic angle of the rotor. B) The decay of two different water signals found in a 1N methanol solution of an AEM membrane with increasing gradient strength. Gradient strength values (G/cm) are shown above the stack plot.

//////
13C-NMR spectroscopy
The basics of 13C-NMR spectroscopy
The magnetic moment of a 13C nucleus is much weaker than that of a proton, meaning that NMR signals from 13C nuclei are inherently much weaker than proton signals. This, combined with the low natural abundance of 13C, means that it is much more difficult to observe carbon signals: more sample is required, and often the data from hundreds of scans must be averaged in order to bring the signal-to-noise ratio down to acceptable levels. Unlike 1H-NMR signals, the area under a 13C-NMR signal cannot be used to determine the number of carbons to which it corresponds. This is because the signals for some types of carbons are inherently weaker than for other types – peaks corresponding to carbonyl carbons, for example, are much smaller than those for methyl or methylene (CH2) peaks. Peak integration is generally not useful in 13C-NMR spectroscopy, except when investigating molecules that have been enriched with 13C isotope (see section 5.6B).
The resonance frequencies of 13C nuclei are lower than those of protons in the same applied field - in a 7.05 Tesla instrument, protons resonate at about 300 MHz, while carbons resonate at about 75 MHz. This is fortunate, as it allows us to look at 13C signals using a completely separate 'window' of radio frequencies. Just like in 1H-NMR, the standard used in 13C-NMR experiments to define the 0 ppm point is tetramethylsilane (TMS), although of course in 13C-NMR it is the signal from the four equivalent carbons in TMS that serves as the standard. Chemical shifts for 13C nuclei in organic molecules are spread out over a much wider range than for protons – up to 200 ppm for 13C compared to 12 ppm for protons This is also fortunate, because it means that the signal from each carbon in a compound can almost always be seen as a distinct peak, without the overlapping that often plagues 1H-NMR spectra. The chemical shift of a 13C nucleus is influenced by essentially the same factors that influence a proton's chemical shift: bonds to electronegative atoms and diamagnetic anisotropy effects tend to shift signals downfield (higher resonance frequency). In addition, sp2 hybridization results in a large downfield shift. The 13C-NMR signals for carbonyl carbons are generally the furthest downfield (170-220 ppm), due to both sp2 hybridization and to the double bond to oxygen.
Because of the low natural abundance of 13C nuclei, it is very unlikely to find two 13C atoms near each other in the same molecule, and thus we do not see spin-spin coupling between neighboring carbons in a 13C-NMR spectrum. There is, however, heteronuclear coupling between 13C carbons and the hydrogens to which they are bound. Carbon-proton coupling constants are very large, on the order of 100 – 250 Hz. For clarity, chemists generally use a technique called broadband decoupling, which essentially 'turns off' C-H coupling, resulting in a spectrum in which all carbon signals are singlets. Below is the proton-decoupled13C-NMR spectrum of ethyl acetate, showing the expected four signals, one for each of the carbons.
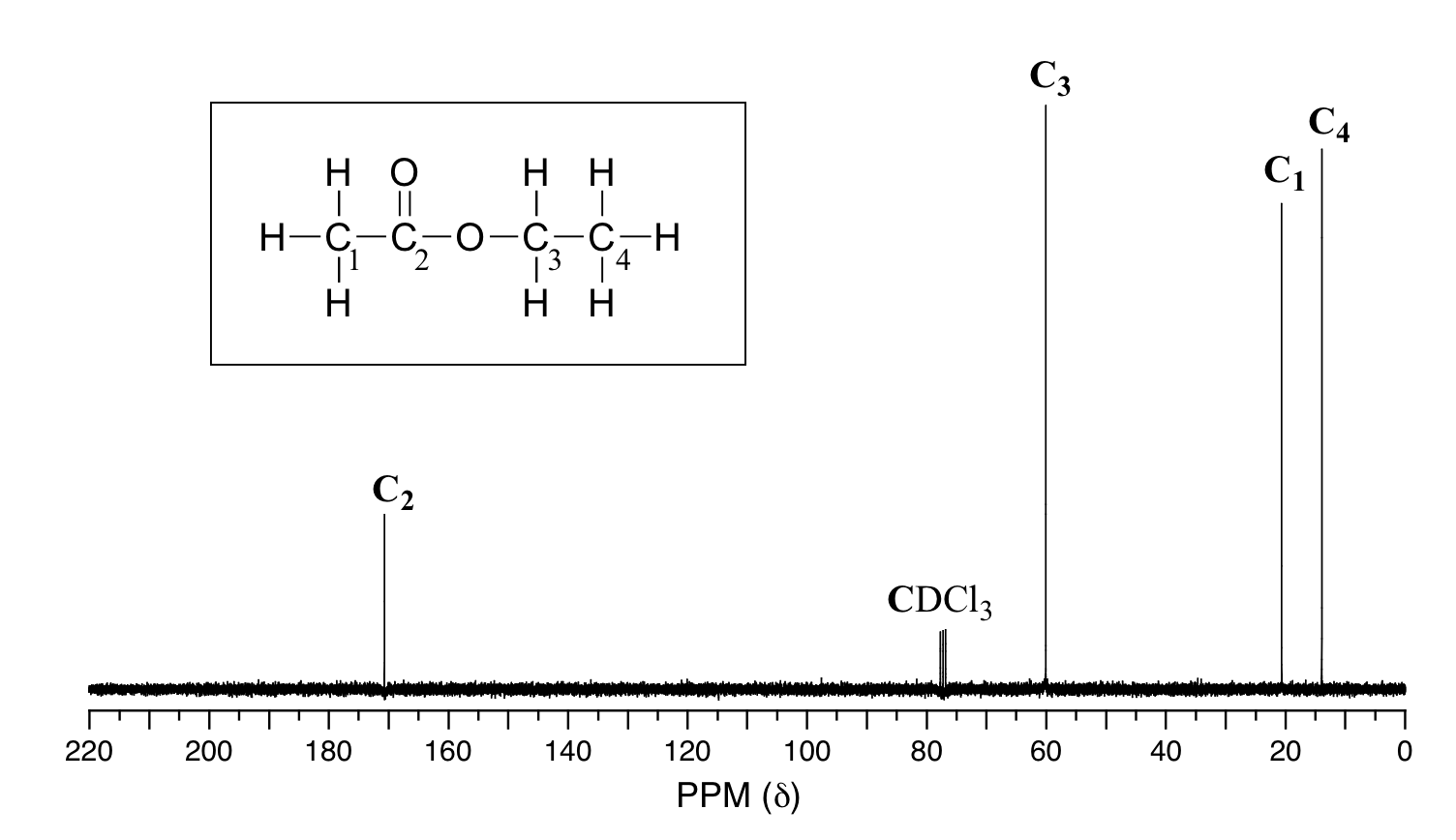
While broadband decoupling results in a much simpler spectrum, useful information about the presence of neighboring protons is lost. However, another modern NMR technique called DEPT (Distortionless Enhancement by Polarization Transfer) allows us to determine how many hydrogens are bound to each carbon. For example, a DEPT experiment tells us that the signal at 171 ppm in the ethyl acetate spectrum is a quaternary carbon (no hydrogens bound, in this case a carbonyl carbon), that the 61 ppm signal is from a methylene (CH2) carbon, and that the 21 ppm and 14 ppm signals are both methyl (CH3) carbons. The details of the DEPT experiment are beyond the scope of this text, but DEPT information will often be provided along with 13C spectral data in examples and problems.
Below are two more examples of 13C NMR spectra of simple organic molecules, along with DEPT information.
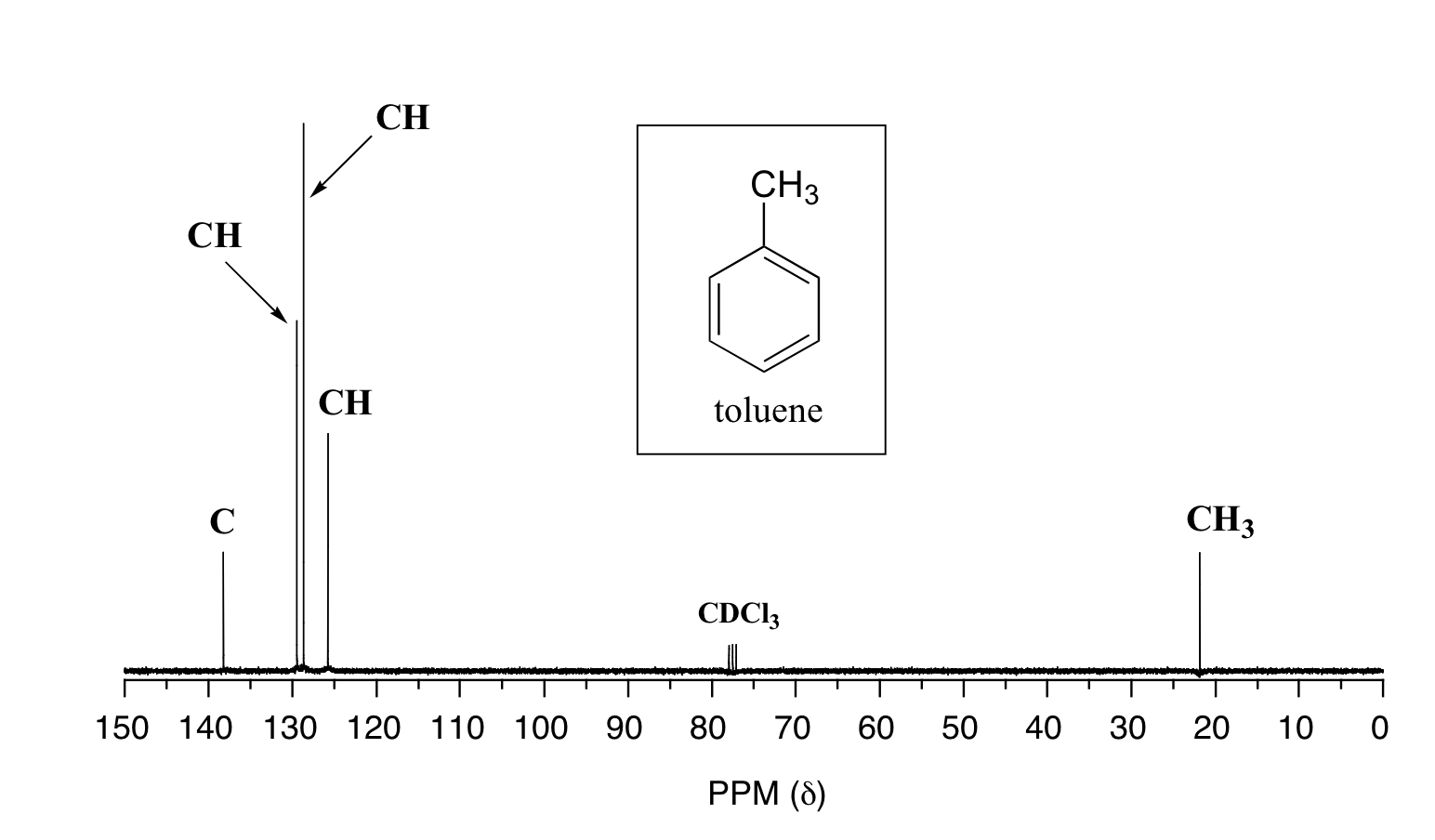

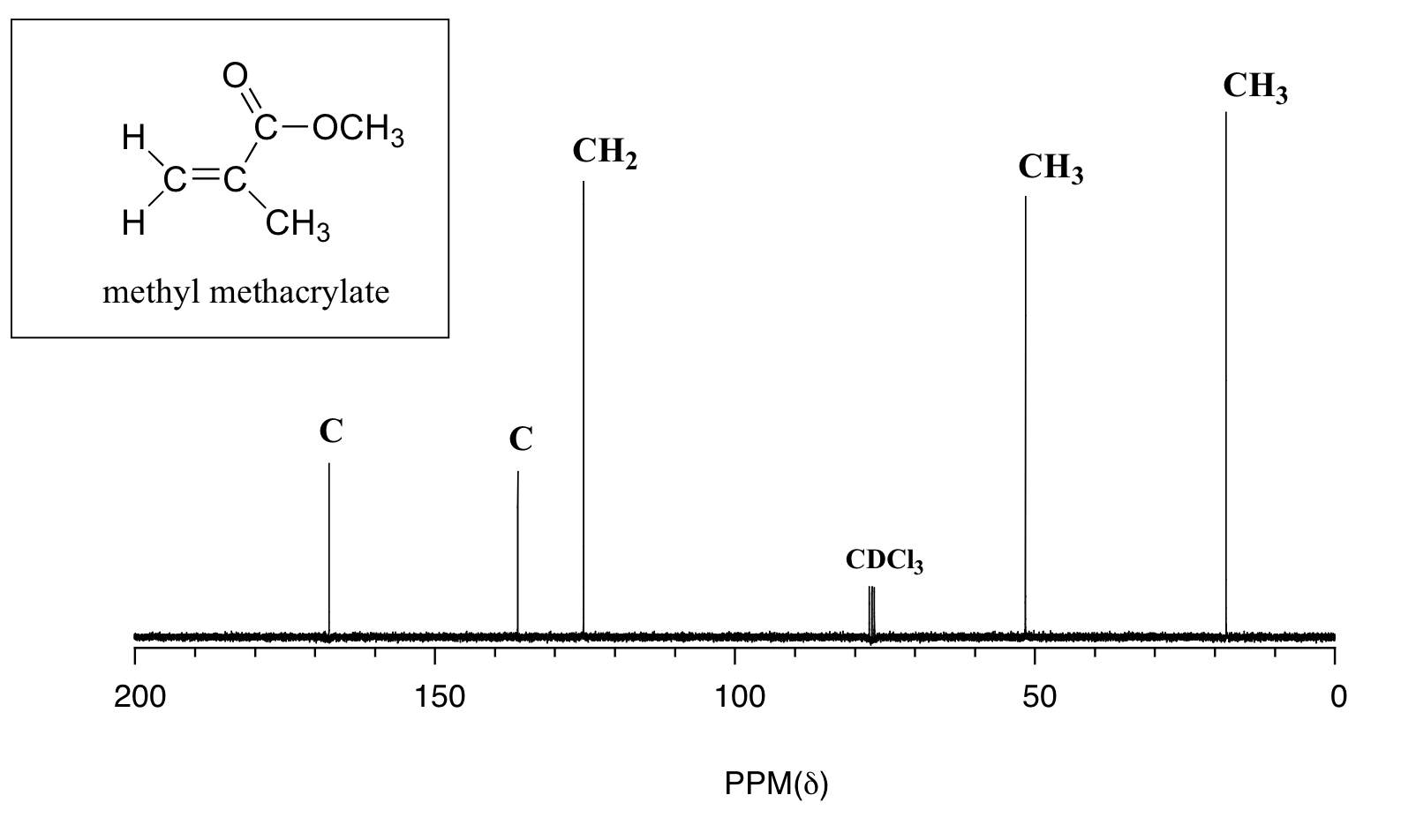
One of the greatest advantages of 13C-NMR compared to 1H-NMR is the breadth of the spectrum - recall that carbons resonate from 0-220 ppm relative to the TMS standard, as opposed to only 0-12 ppm for protons. Because of this, 13C signals rarely overlap, and we can almost always distinguish separate peaks for each carbon, even in a relatively large compound containing carbons in very similar environments. In the proton spectrum of 1-heptanol, for example, only the signals for the alcohol proton (Ha) and the two protons on the adjacent carbon (Hb) are easily analyzed. The other proton signals overlap, making analysis difficult.
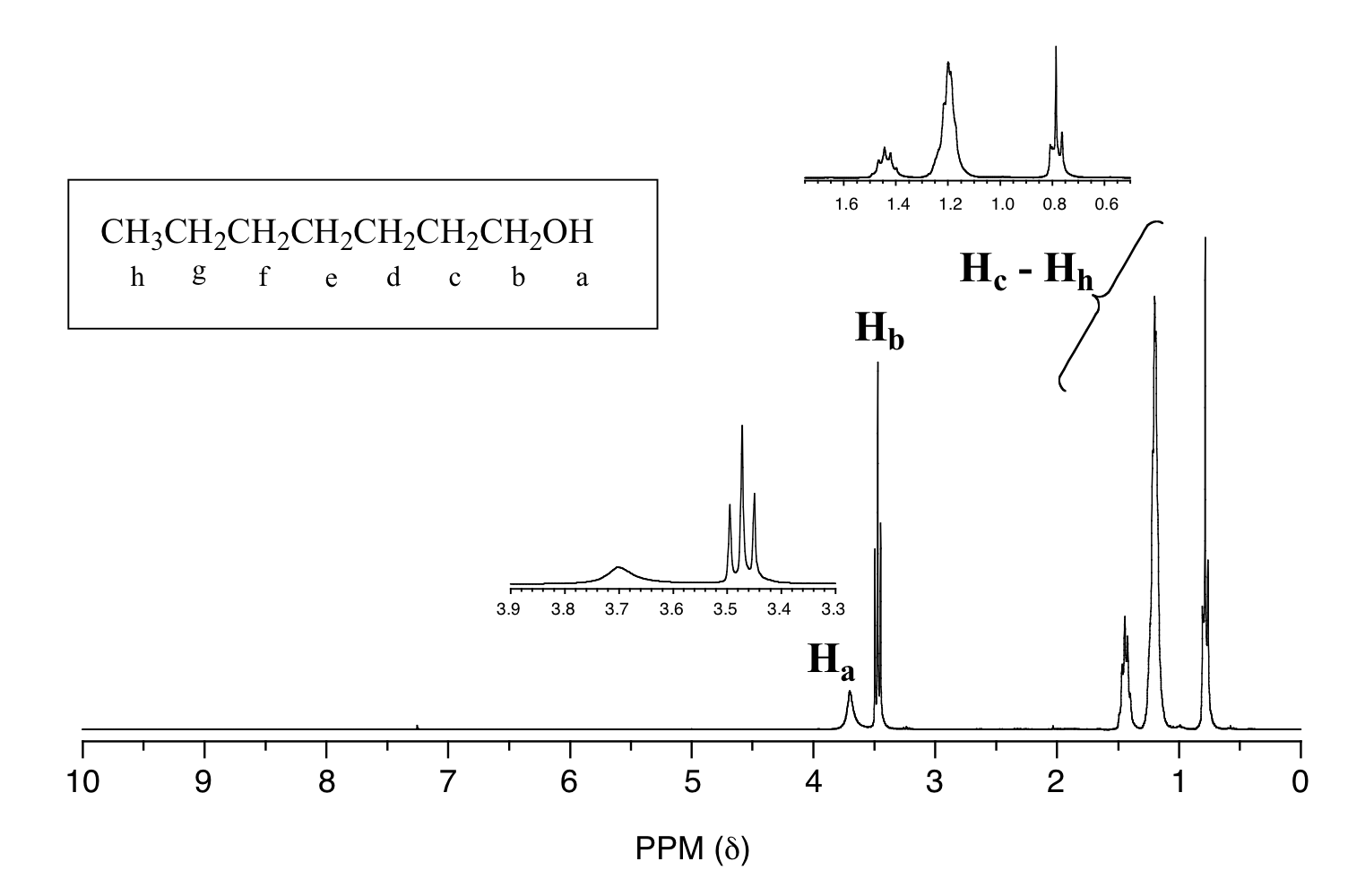
In the 13C spectrum of the same molecule, however, we can easily distinguish each carbon signal, and we know from this data that our sample has seven non-equivalent carbons. (Notice also that, as we would expect, the chemical shifts of the carbons get progressively smaller as they get farther away from the deshielding oxygen.)
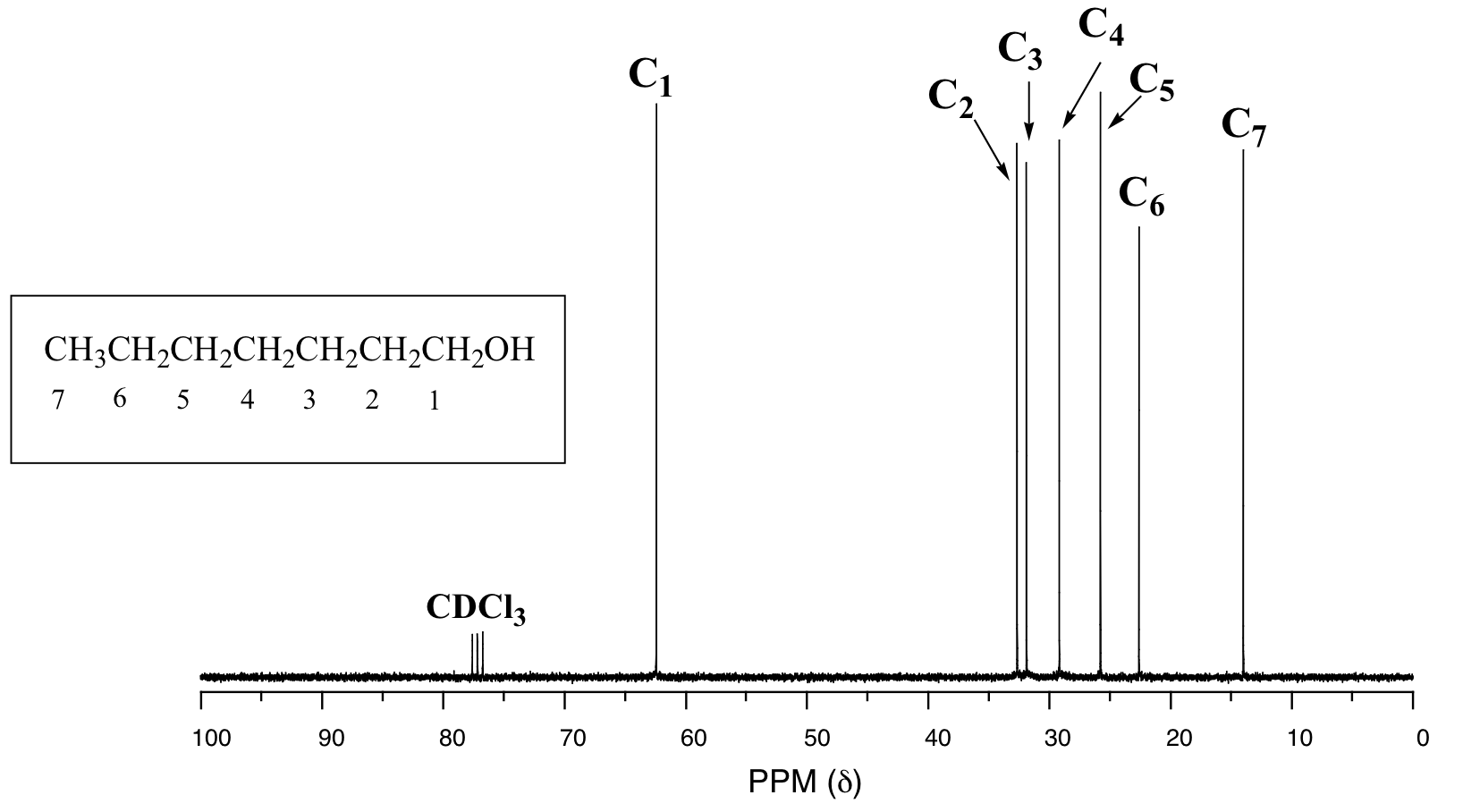
This property of 13C-NMR makes it very helpful in the elucidation of larger, more complex structures.
//////////////////////
Infrared (IR) spectroscopy
Infrared (IR) spectroscopy
In organic compounds, atoms are said to be bonded to each other through a σ bond when the two bonded atoms are held together by mutual attraction for the shared electron pair that lies between them. The two atoms do not remain static at a fixed distance from one another, however. They are free to vibrate back and forth about an average separation distance known as the average bond length. These movements are termed stretching vibrations. In addition, the bond axis (defined as the line directly joining two bonded atoms) of one bond may rock back and forth within the plane it shares with another bond or bend back and forth outside that plane. These movements are called bending vibrations. Both stretching and bending vibrations represent different energy levels of a molecule. These energy differences match the energies of wavelengths in the infrared region of the electromagnetic spectrum—i.e., those ranging from 2.5 to 15 micrometres (μm; 1 μm = 10−6m). An infrared spectrophotometer is an instrument that passes infrared light through an organic molecule and produces a spectrum that contains a plot of the amount of light transmitted on the vertical axis against the wavelength of infrared radiation on the horizontal axis. In infrared spectra the absorption peaks point downward because the vertical axis is the percent transmittance of the radiation through the sample. Absorption of radiation lowers the percent transmittance value. Since all bonds in an organic molecule interact with infrared radiation, IR spectra provide a great deal of structural data.
- Stretching and bending vibrations in organic compounds such as 5-hexene-2-one represent different …Encyclopædia Britannica, Inc.
The stretching vibrations of strong carbon-hydrogen bonds cause the absorptions around 3.4 μm, with the sharp peak at 3.2 μm due to the hydrogen atom on the carbon-carbon double bond. The many bending vibrations of carbon-hydrogen bonds cause the complicated absorption pattern ranging from about 7 to 25 μm. This area of IR spectra is called the fingerprint region, because the absorption pattern is highly complex but unique to each organic structure. The stretching vibrations for both the carbon-carbon and carbon-oxygen double bonds are easily identified at 6.1 and 5.8 μm, respectively. Most of the functional groups have characteristic IR absorptions similar to those for carbon-oxygen and carbon-carbon double bonds. Infrared spectroscopy is therefore extremely useful for determining the types of functional groups present in organic molecules.
/////////////
https://www.britannica.com/science/chemical-compound/Spectroscopy-of-organic-compounds
WHAT IS AN INFRA-RED SPECTRUM?
This page describes what an infra-red spectrum is and how it arises from bond vibrations within organic molecules.
The background to infra-red spectroscopyHow an infra-red spectrum is produced You probably know that visible light is made up of a continuous range of different electromagnetic frequencies - each frequency can be seen as a different colour. Infra-red radiation also consists of a continuous range of frequencies - it so happens that our eyes can't detect them. If you shine a range of infra-red frequencies one at a time through a sample of an organic compound, you find that some frequencies get absorbed by the compound. A detector on the other side of the compound would show that some frequencies pass through the compound with almost no loss, but other frequencies are strongly absorbed. How much of a particular frequency gets through the compound is measured as percentage transmittance. A percentage transmittance of 100 would mean that all of that frequency passed straight through the compound without any being absorbed. In practice, that never happens - there is always some small loss, giving a transmittance of perhaps 95% as the best you can achieve. A transmittance of only 5% would mean that nearly all of that particular frequency is absorbed by the compound. A very high absorption of this sort tells you important things about the bonds in the compound. What an infra-red spectrum looks like A graph is produced showing how the percentage transmittance varies with the frequency of the infra-red radiation. | |
Note: The infra-red spectra on this page have been produced from graphs taken from the Spectral Data Base System for Organic Compounds (SDBS) at the National Institute of Materials and Chemical Research in Japan.It is possible that small errors may have been introduced during the process of converting them for use on this site, but these won't affect the argument in any way. | |
Notice that an unusual measure of frequency is used on the horizontal axis. Wavenumber is defined like this: Similarly, don't worry about the change of scale half-way across the horizontal axis. You will find infra-red spectra where the scale is consistent all the way across, infra-red spectra where the scale changes at around 2000 cm-1, and very occasionally where the scale changes again at around 1000 cm-1. As you will see when we look at how to interpret infra-red spectra, this doesn't cause any problems - you simply need to be careful reading the horizontal scale. What causes some frequencies to be absorbed? Each frequency of light (including infra-red) has a certain energy. If a particular frequency is being absorbed as it passes through the compound being investigated, it must mean that its energy is being transferred to the compound. Energies in infra-red radiation correspond to the energies involved in bond vibrations. Bond stretching In covalent bonds, atoms aren't joined by rigid links - the two atoms are held together because both nuclei are attracted to the same pair of electrons. The two nuclei can vibrate backwards and forwards - towards and away from each other - around an average position. The diagram shows the stretching that happens in a carbon-oxygen single bond. There will, of course, be other atoms attached to both the carbon and the oxygen. For example, it could be the carbon-oxygen bond in methanol, CH3OH. Bonds are vibrating all the time, but if you shine exactly the right amount of energy on a bond, you can kick it into a higher state of vibration. The amount of energy it needs to do this will vary from bond to bond, and so each different bond will absorb a different frequency (and hence energy) of infra-red radiation. Bond bending As well as stretching, bonds can also bend. The diagram shows the bending of the bonds in a water molecule. The effect of this, of course, is that the bond angle between the two hydrogen-oxygen bonds fluctuates slightly around its average value. Imagine a lab model of a water molecule where the atoms are joined together with springs. These bending vibrations are what you would see if you shook the model gently. Tying all this together Look again at the infra-red spectrum of propan-1-ol, CH3CH2CH2OH: |
INTERPRETING AN INFRA-RED SPECTRUM This page explains how to use an infra-red spectrum to identify the presence of a few simple bonds in organic compounds. | |
The infra-red spectrum for a simple carboxylic acidEthanoic acid Ethanoic acid has the structure:
The carbon-oxygen single bond also has an absorbtion in the fingerprint region, varying between 1000 and 1300 cm-1depending on the molecule it is in. You have to be very wary about picking out a particular trough as being due to a C-O bond. | |
The other bonds in ethanoic acid have easily recognised absorptions outside the fingerprint region. The C-H bond (where the hydrogen is attached to a carbon which is singly-bonded to everything else) absorbs somewhere in the range from 2853 - 2962 cm-1. Because that bond is present in most organic compounds, that's not terribly useful! What it means is that you can ignore a trough just under 3000 cm-1, because that is probably just due to C-H bonds. The carbon-oxygen double bond, C=O, is one of the really useful absorptions, found in the range 1680 - 1750 cm-1. Its position varies slightly depending on what sort of compound it is in. The other really useful bond is the O-H bond. This absorbs differently depending on its environment. It is easily recognised in an acid because it produces a very broad trough in the range 2500 - 3300 | |
The infra-red spectrum for ethanoic acid looks like this: | |
The infra-red spectrum for an alcoholEthanol Notice the absorption due to the C-H bonds just under 3000 cm-1, and also the troughs between 1000 and 1100 cm-1 - one of which will be due to the C-O bond. The infra-red spectrum for an esterEthyl ethanoate The C-O single bond is the absorption at about 1240 cm-1. Whether or not you could pick that out would depend on the detail given by the table of data which you get in your exam, because C-O single bonds vary anywhere between 1000 and 1300 cm-1 depending on what sort of compound they are in. Some tables of data fine it down, so that they will tell you that an absorption from 1230 - 1250 is the C-O bond in an ethanoate. The infra-red spectrum for a ketonePropanone Confusingly, there are also absorptions which look as if they might be due to C-O single bonds - which, of course, aren't present in propanone. This reinforces the care you have to take in trying to identify any absorptions in the fingerprint region. Aldehydes will have similar infra-red spectra to ketones. The infra-red spectrum for a hydroxy-acid2-hydroxypropanoic acid (lactic acid) The O-H bond in the acid group absorbs between 2500 and 3300, the one in the chain between 3230 and 3550 cm-1. Taken together, that gives this immense trough covering the whole range from 2500 to 3550 cm-1. Lost in that trough as well will be absorptions due to the C-H bonds. Notice also the presence of the strong C=O absorption at about 1730 cm-1. The infra-red spectrum for a primary amine1-aminobutane |
THE FINGERPRINT REGION OF AN INFRA-RED SPECTRUM This page explains what the fingerprint region of an infra-red spectrum is, and how it can be used to identify an organic molecule. | |
What is the fingerprint region This is a typical infra-red spectrum: | |
Each trough is caused because energy is being absorbed from that particular frequency of infra-red radiation to excite bonds in the molecule to a higher state of vibration - either stretching or bending. Some of the troughs are easily used to identify particular bonds in a molecule. For example, the big trough at the left-hand side of the spectrum is used to identify the presence of an oxygen-hydrogen bond in an -OH group. | |
The region to the right-hand side of the diagram (from about 1500 to 500 cm-1) usually contains a very complicated series of absorptions. These are mainly due to all manner of bending vibrations within the molecule. This is called the fingerprint region. It is much more difficult to pick out individual bonds in this region than it is in the "cleaner" region at higher wavenumbers. The importance of the fingerprint region is that each different compound produces a different pattern of troughs in this part of the spectrum. Using the fingerprint region Compare the infra-red spectra of propan-1-ol and propan-2-ol. Both compounds contain exactly the same bonds. Both compounds have very similar troughs in the area around 3000 cm-1 - but compare them in the fingerprint region between 1500 and 500 cm-1. So . . . to positively identify an unknown compound, use its infra-red spectrum to identify what sort of compound it is by looking for specific bond absorptions. That might tell you, for example, that you had an alcohol because it contained an -OH group. You would then compare the fingerprint region of its infra-red spectrum with known spectra measured under exactly the same conditions to find out which alcohol (or whatever) you had. |
Friday, 27 October 2017
1-(Ferrocenyl(phenyl)methyl)pyrrolidine
1-(Ferrocenyl(phenyl)methyl)pyrrolidine (1b) was obtained (2.14 g, 62% yield) as an orange solid. m.p. 100-102 oC;
1H NMR (400 MHz, CDCl3) δ 7.53 (d, J = 7.6 Hz, 2H), 7.38 (t, J = 7.6 Hz, 2H), 7.29 (t, J = 7.6 Hz, 1H), 4.18 (s, 2H), 4.12 (s, 1H), 4.07 (s, 1H), 3.80 (s, 1H), 3.74 (s, 5H), 2.32 (s, 4H), 1.68 (s, 4H);
13C NMR (100 MHz, CDCl3) δ 128.2, 127.9, 127.0, 71.2, 69.9, 68.6, 68.5, 67.2, 66.4, 54.0, 23.2;
HRMS (ESI) calcd for C21H24FeN+ (M + H)+ 346.1253, found 346.1247.
////////////
Wednesday, 25 October 2017
Cross dehydrogenative coupling of N-aryltetrahydroisoquinolines (sp3 C-H) with indoles (sp2 C-H) using a heterogeneous mesoporous manganese oxide catalyst
Cross dehydrogenative coupling of N-aryltetrahydroisoquinolines (sp3 C-H) with indoles (sp2 C-H) using a heterogeneous mesoporous manganese oxide catalyst
Green Chem., 2017, Advance Article
DOI: 10.1039/C7GC01919J, Communication
DOI: 10.1039/C7GC01919J, Communication
B. Dutta, V. Sharma, N. Sassu, Y. Dang, C. Weerakkody, J. Macharia, R. Miao, A. R. Howell, S. L. Suib
We disclose a novel, heterogeneous catalytic approach for selective coupling of C1 of N-aryltetrahydroisoquinolines with C3 of indoles in the presence of mesoporous manganese oxides.
We disclose a novel, heterogeneous catalytic approach for selective coupling of C1 of N-aryltetrahydroisoquinolines with C3 of indoles in the presence of mesoporous manganese oxides.
Cross dehydrogenative coupling of N-aryltetrahydroisoquinolines (sp3 C–H) with indoles (sp2 C–H) using a heterogeneous mesoporous manganese oxide catalyst
B. Dutta,a V. Sharma,b N. Sassu,a Y. Dang,c C. Weerakkody,a J. Macharia,a R. Miao,a A. R. Howella and S. L. Suib*ac
*Corresponding authors
bMaterials Science and Technology Division, Oak Ridge National Laboratory, Oak Ridge, USA
cInstitute of Materials Science, University of Connecticut, U-3060, 55 North Eagleville Rd., Storrs, USA
Biswanath Dutta
Ph.D Candidate
Chemistry
B.Sc. Chemistry, University of Calcutta, India, 2011
M.S. Chemistry, IIT Bombay, India, 2013
Group Member Since 2013
Research Area: Material synthesis, Catalysis
Steven Suib
Professor
steven.suib@uconn.edu | |
Phone | (860) 486-2797 |
Fax | (860) 486-2981 |
Mailing Address | University of Connecticut Department of Chemistry 55 N. Eagleville Rd Storrs, CT 06269 |
Office Location | CHEM A-313 |
Abstract
We disclose a novel, heterogeneous catalytic approach for selective coupling of C1 of N-aryltetrahydroisoquinolines with C3 of indoles in the presence of mesoporous manganese oxides. Our work involves a detailed mechanistic investigation of the reaction on the catalyst surface, backed by DFT computational studies, to understand the superior catalytic activity of manganese oxides.
////////////////////
Subscribe to:
Posts (Atom)