Nobilisitine A was isolated by Evidente and coworkers, who proposed the structure 1.1 Banwell and co-workers then synthesized the enantiomer of 1, but its NMR did not correspond to that of reported for Nobilisitine A.; the largest differences are 4.7 ppm for the 13C NMR and 0.79 ppm for the 1H NMR.2
1
Lodewyk and Tantillo3 examined seven diastereomers of 1, all of which have a cis fusion
between the saturated 5 and six-member rings (rings C and D). Low
energy conformations were computed for each of these diasteromers at
B3LYP/6-31+G(d,p). NMR shielding constants were then computed in solvent
(using a continuum approach) at mPW1PW91/6-311+G(2d,p). A Boltzmann
weighting of the shielding contants was then computed, and these shifts
were then scaled as described by Jain, Bally and Rablen4 (discussed in this post). The computed NMR shifts for 1 were compared with the experimental values, and the mean deviations for the 13C and 1H svalues is 1.2 and 0.13 ppm, respectively. (The largest outlier is 3.4 ppm for 13C and 0.31 for 1H
shifts.) Comparison was then made between the computed shifts of the
seven diasteomers and the reported spectrum of Nobilisitine A, and the
lowest mean deviations (1.4 ppm for 13C and 0.21 ppm for 1H) is for structure 2. However, the agreement is not substantially better than for a couple of the other diasteomers.
2
They next employed the DP4 analysis developed by Smith and Goodman5 for just such a situation – where you have an experimental spectrum and a number of potential diastereomeric structures. (See this post for a discussion of the DP4 method.)The DP4 analysis suggests that 2 is the correct structure with a probability of 99.8%.
Banwell has now synthesized the compound with structure 2 and its NMR matches that of the original natural product.6 Thus Nobilisitine A has the structure 2.
References
(1)
Evidente, A.; Abou-Donia, A. H.; Darwish, F. A.; Amer, M. E.; Kassem,
F. F.; Hammoda, H. A. m.; Motta, A., "Nobilisitine A and B, two
masanane-type alkaloids from Clivia nobilis,"Phytochemistry, 1999, 51, 1151-1155, DOI: 10.1016/S0031-9422(98)00714-6.
(2)
Schwartz, B. D.; Jones, M. T.; Banwell, M. G.; Cade, I. A., "Synthesis
of the Enantiomer of the Structure Assigned to the Natural Product
Nobilisitine A," Org. Lett., 2010, 12, 5210-5213, DOI:10.1021/ol102249q
(3) Lodewyk, M. W.; Tantillo, D. J., "Prediction of the Structure of Nobilisitine A Using Computed NMR Chemical Shifts," J. Nat. Prod., 2011, 74, 1339-1343, DOI: 10.1021/np2000446
(4)
Jain, R.; Bally, T.; Rablen, P. R., "Calculating Accurate Proton
Chemical Shifts of Organic Molecules with Density Functional Methods and
Modest Basis Sets," J. Org. Chem., 2009, DOI:10.1021/jo900482q.
(5)
Smith, S. G.; Goodman, J. M., "Assigning Stereochemistry to Single
Diastereoisomers by GIAO NMR Calculation: The DP4 Probability," J. Am. Chem. Soc., 2010, 132, 12946-12959, DOI:10.1021/ja105035r
(6) Schwartz, B. D.; White, L. V.; Banwell, M. G.; Willis, A. C., "Structure of the Lycorinine Alkaloid Nobilisitine A," J. Org. Chem., 2011, ASAP, DOI: 10.1021/jo2016899
a tour
MYSORE, KARNATAKA, INDIA


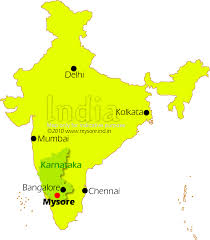



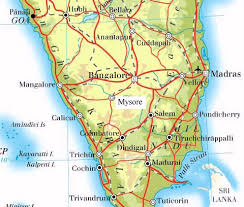



















////////////
a tour
MYSORE, KARNATAKA, INDIA














////////////