Organic Chemists from Industry and academics to Interact on Spectroscopy Techniques for Organic Compounds ie NMR, MASS, IR, UV Etc. Starters, Learners, advanced, all alike, contains content which is basic or advanced, by Dr Anthony Melvin Crasto, Worlddrugtracker, email me ........... amcrasto@gmail.com, call +91 9323115463 India skype amcrasto64
................DR ANTHONY MELVIN CRASTO Ph.D ( ICT, Mumbai) , INDIA 25Yrs Exp. in the feld of Organic Chemistry,Working for GLENMARK GENERICS at Navi Mumbai, INDIA. Serving chemists around the world. Helping them with websites on Chemistry.Million hits on google, world acclamation from industry, academia, drug authorities for websites, blogs and educational contribution
Pages
- Home
- ABOUT ME
- DIMENSIONS IN NMR SPECTROSCOPY
- 13 C NMR
- 1H NMR
- CHEMDOODLE/INTERACTIVE SPECT PREDICT
- Animations
- HELP ME
- Multinuclear NMR Spectroscopy
- Examples of 13C NMR
- Books on NMR spectroscopy
- UV-Visible Spectroscopy
- IR SPECTRA EXAMPLES
- Journals
- Organic spectroscopy site
- Spectroscopy sites
- IR SPECTROSCOPY
- Books-2
- Recommended Web Sites for Spectra and Spectrum-rel...
- DISCLAIMER
- Mössbauer spectroscopy
- FINDING CHEMICAL SPECTRA
- Mass Spectrometry
- NMR Overview
- Characterisation of Organic Compounds
- SDBS Spectral Database System for Organic Compounds
- CHEMICAL SHIFT
- MASS SPECTROSCOPY
- Books-1
- MASSBANK PORTAL
- 11B NMR
Saturday 31 October 2015
Malleobactin Structure Elucidated
A team of scientists based in Germany have elucidated the structure and absolute configuration of malleobactin, which is the siderophore of the pathogenic Burkholderia mallei. Siderophores are strong iron-binding agents, and act as virulence factors for pathogenic bacteria.
Read more
http://www.chemistryviews.org/details/ezine/4997511/Malleobactin_Structure_Elucidated.html
TALE A TOUR
Isle Of Capri, Italy.























/////////
Computed NMR spectra predicts the structure of Nobilisitine A
Nobilisitine A was isolated by Evidente and coworkers, who proposed the structure 1.1 Banwell and co-workers then synthesized the enantiomer of 1, but its NMR did not correspond to that of reported for Nobilisitine A.; the largest differences are 4.7 ppm for the 13C NMR and 0.79 ppm for the 1H NMR.2
1
Lodewyk and Tantillo3 examined seven diastereomers of 1, all of which have a cis fusion
between the saturated 5 and six-member rings (rings C and D). Low
energy conformations were computed for each of these diasteromers at
B3LYP/6-31+G(d,p). NMR shielding constants were then computed in solvent
(using a continuum approach) at mPW1PW91/6-311+G(2d,p). A Boltzmann
weighting of the shielding contants was then computed, and these shifts
were then scaled as described by Jain, Bally and Rablen4 (discussed in this post). The computed NMR shifts for 1 were compared with the experimental values, and the mean deviations for the 13C and 1H svalues is 1.2 and 0.13 ppm, respectively. (The largest outlier is 3.4 ppm for 13C and 0.31 for 1H
shifts.) Comparison was then made between the computed shifts of the
seven diasteomers and the reported spectrum of Nobilisitine A, and the
lowest mean deviations (1.4 ppm for 13C and 0.21 ppm for 1H) is for structure 2. However, the agreement is not substantially better than for a couple of the other diasteomers.
2
They next employed the DP4 analysis developed by Smith and Goodman5 for just such a situation – where you have an experimental spectrum and a number of potential diastereomeric structures. (See this post for a discussion of the DP4 method.)The DP4 analysis suggests that 2 is the correct structure with a probability of 99.8%.
Banwell has now synthesized the compound with structure 2 and its NMR matches that of the original natural product.6 Thus Nobilisitine A has the structure 2.
References
(1)
Evidente, A.; Abou-Donia, A. H.; Darwish, F. A.; Amer, M. E.; Kassem,
F. F.; Hammoda, H. A. m.; Motta, A., "Nobilisitine A and B, two
masanane-type alkaloids from Clivia nobilis,"Phytochemistry, 1999, 51, 1151-1155, DOI: 10.1016/S0031-9422(98)00714-6.
(2)
Schwartz, B. D.; Jones, M. T.; Banwell, M. G.; Cade, I. A., "Synthesis
of the Enantiomer of the Structure Assigned to the Natural Product
Nobilisitine A," Org. Lett., 2010, 12, 5210-5213, DOI:10.1021/ol102249q
(3) Lodewyk, M. W.; Tantillo, D. J., "Prediction of the Structure of Nobilisitine A Using Computed NMR Chemical Shifts," J. Nat. Prod., 2011, 74, 1339-1343, DOI: 10.1021/np2000446
(4)
Jain, R.; Bally, T.; Rablen, P. R., "Calculating Accurate Proton
Chemical Shifts of Organic Molecules with Density Functional Methods and
Modest Basis Sets," J. Org. Chem., 2009, DOI:10.1021/jo900482q.
(5)
Smith, S. G.; Goodman, J. M., "Assigning Stereochemistry to Single
Diastereoisomers by GIAO NMR Calculation: The DP4 Probability," J. Am. Chem. Soc., 2010, 132, 12946-12959, DOI:10.1021/ja105035r
(6) Schwartz, B. D.; White, L. V.; Banwell, M. G.; Willis, A. C., "Structure of the Lycorinine Alkaloid Nobilisitine A," J. Org. Chem., 2011, ASAP, DOI: 10.1021/jo2016899
Absolute Configuration of (+)-Erythro-Mefloquine
The absolute configuration of (+)-erythro-mefloquine has been confirmed by X-ray crystallography, CD spectroscopy, and molecular modeling
Read more
http://www.chemistryviews.org/details/ezine/4948391/Absolute_Configuration_of_-Erythro-Mefloquine.html
Alexandra Dassonville-Klimpt, Christine Cézard, Catherine Mullié, Patrice Agnamey, Alexia Jonet, Sophie Da Nascimento, Mathieu Marchivie, Jean Guillon, Pascal Sonnet,
ChemPlusChem 2013.
DOI: 10.1002/cplu.201300074
Michael Müller, Claudia M. Orben, Nina Schützenmeister, Manuel Schmidt, Andrei Leonov, Uwe M. Reinscheid, Birger Dittrich, Christian Griesinger,
Angew. Chem. Int. Ed. 2013.
DOI: 10.1002/anie.201300258
1-Adamantyl cation – Predicting its NMR spectra
1-Adamantyl cation – Predicting its NMR spectra
What is required in order to compute very accurate
NMR chemical shifts? Harding, Gauss and Schleyer take on the
interesting spectrum of 1-adamantyl cation to try to discern the
important factors in computing its 13C and 1H chemical shifts.1
1
To start, the chemical shifts of 1-adamtyl cation were computed at B3LYP/def2-QZVPP and
MP2/qz2p//MP2/cc-pVTZ. The root means square error (compared to experiment) for the carbon chemical shifts is large: 12.76 for B3LYP and 6.69 for MP2. The proton shifts are predicted much more accurately with an RMS error of 0.27 and 0.19 ppm, respectively.
MP2/qz2p//MP2/cc-pVTZ. The root means square error (compared to experiment) for the carbon chemical shifts is large: 12.76 for B3LYP and 6.69 for MP2. The proton shifts are predicted much more accurately with an RMS error of 0.27 and 0.19 ppm, respectively.
The authors speculate that the underlying cause of the poor prediction is the geometry of the molecule. The structure of 1 was
optimized at HF/cc-pVTZ, MP2/cc-pVTZ and CCSD(T)/pVTZ and then the
chemical shifts were computed using MP2/tzp with each optimized
geometry. The RMS error of the 12C
chemical shifts are HF/cc-pVTZ: 9.55, MP2/cc-pVTZ: 5.62, and
CCSD(T)/pVTZ: 5.06. Similar relationship is seen in the proton chemical
shifts. Thus, a better geometry does seem to matter. The CCSD(T)/pVTZ
optimized structure of 1 is shown in Figure 1.
1
|
Figure 1. CCSD(T)/pVTZ optimized structure of 1.
Unfortunately,
the computed chemical shifts at CCSD(T)/qz2p//CCSD(T)/cc-pVTZ are still
in error; the RMS is 4.78ppm for the carbon shifts and 0.26ppm for the
proton shifts. Including a correction for the zero-point vibrational
effects and adjusting to a temperature of 193 K to match the experiment
does reduce the error; now the RMS for the carbon shifts is 3.85 ppm,
with the maximum error of 6 ppm for C3. The RMS for the proton chemical
shifts is 0.21ppm.
The
remaining error they attribute to basis set incompleteness in the NMR
computation, a low level treatment of the zero-point vibrational effects
(which were computed at HF/tz2p), neglect of the solvent, and use of a
reference in the experiment that was not dissolved in the same media as
the adamantyl cation.
So,
to answer our opening question – it appears that a very good geometry
and treatment of vibrational effects is critical to accurate NMR shift
computation of this intriguing molecule. Let the
computational chemist beware!
computational chemist beware!
References
(1)
Harding, M. E.; Gauss, J.; Schleyer, P. v. R., "Why Benchmark-Quality
Computations Are Needed To Reproduce 1-Adamantyl Cation NMR Chemical
Shifts Accurately," J. Phys. Chem. A, 2011, 115, 2340-2344, DOI: 10.1021/jp1103356
//////////
Welwitindolinones structure
A
quick note here on the use of computed NMR to determine stereochemical
structure. The Garg group synthesized two “oxidized welwitindolines”,
compounds 1 and 2.1 The relative stereochemistry at the C3 position (the carbon with the hydroxy group) was unknown.
1 | 2 |
Low energy gas-phase conformers of both epimers of 1 and 2 were
optimized at B3LYP/6-31+G(d,p). (These computations were done by the
Tantillo group.) See Figure 1 for the optimized lowest energy
conformers. Using these geometries the NMR chemical shifts were computed
at mPW1PW91/6-311+G(d,p) with implicit solvent (chloroform). The
chemical shifts were Boltzmann-weighted and scaled according to the
prescription (see this post) of Jain, Bally and Rablen.2 The computed chemical shifts were then compared against the experimental NMR spectra. For both 1and 2, the 13C NMR shifts could not readily distinguish the two epimers. However, the computed 1H chemical shifts for the S epimer of each compound was significantly in better agreement with the experimental values; the mean average deviation for the S epimer of 2 is 0.08 ppm but 0.36ppm for the R epimer. As a check of these results, DP4 analysis3 (see this post) of 2 indicated a 100% probability for the S epimer using only the proton chemical shifts or with the combination of proton and carbon data.
1
|
2
|
Figure 1. B3LYP/6-31+G(d,p) optimized geometries of the
lowest energy conformations of 1 and 2.
lowest energy conformations of 1 and 2.
References
(1)
Quasdorf, K. W.; Huters, A. D.; Lodewyk, M. W.; Tantillo, D. J.; Garg,
N. K., "Total Synthesis of Oxidized Welwitindolinones and (-)-N-Methylwelwitindolinone C Isonitrile," J. Am. Chem. Soc. 2011,134, 1396-1399, DOI: 10.1021/ja210837b
(2)
Jain, R.; Bally, T.; Rablen, P. R., "Calculating Accurate Proton
Chemical Shifts of Organic Molecules with Density Functional Methods and
Modest Basis Sets," J. Org. Chem. 2009, 74, 4017-4023, DOI: 10.1021/jo900482q.
(3)
Smith, S. G.; Goodman, J. M., "Assigning Stereochemistry to Single
Diastereoisomers by GIAO NMR Calculation: The DP4 Probability," J. Am. Chem. Soc. 2010, 132, 12946-12959, DOI:10.1021/ja105035r
/////
Monday 26 October 2015
Omarigliptin
OMARIGLIPTIN. MK 3102
1226781-44-7
(2R,3S,5R)-2-(2,5-difluorophenyl)-5-[2-(methylsulfonyl)-2,6-dihydropyrrolo[3,4-c]pyrazol-5(4H)-yl]tetrahydro-2H-pyran-3-amine
(2R,3S,5R)-2-(2,5-difluorophenyl)-5-(2-methylsulfonyl-4,6-dihydropyrrolo[3,4-c]pyrazol-5-yl)oxan-3-amine
(2R,3S,5R)-2-(2,5-Difluorophenyl)-5-[2-(methylsulfonyl)-2,6-dihydropyrrolo[3,4-c]pyrazol-5(4H)-yl]tetrahydro-2H-pyran-3-amine
1226781-44-7, Omarigliptin [USAN], UNII-CVP59Q4JE1, CHEMBL2105762, MK-3102, PB39113
Molecular Formula: C17H20F2N4O3S Molecular Weight: 398.427506
IN PHASE 3
CLICK ON IMAGES FOR CLARITY VIEW
PAPER
J. Med. Chem., 2014, 57 (8), pp 3205–3212
DOI: 10.1021/jm401992e
In our effort to discover DPP-4 inhibitors with added benefits over currently commercially available DPP-4 inhibitors, MK-3102 (omarigliptin), was identified as a potent and selective dipeptidyl peptidase 4 (DPP-4) inhibitor with an excellent pharmacokinetic profile amenable for once-weekly human dosing and selected as a clinical development candidate. This manuscript summarizes the mechanism of action, scientific rationale, medicinal chemistry, pharmacokinetic properties, and human efficacy data for omarigliptin, which is currently in phase 3 clinical development.
Omarigliptin (MK-3102) (2R,3S,5R)-2-(2,5-difluorophenyl)-5-[2-(methylsulfonyl)-2,6-dihydropyrrolo[3,4-c]pyrazol-5(4H)-yl]tetrahydro-2H-pyran-3-amine,
is a 2,3,5-substituted tetrahydropyran analogue currently in phase 3 clinical trial for type 2 diabetes mellitus (T2DM).
(2R,3S,5R)-2-(2,5-Difluorophenyl)-5-[2-(methylsulfonyl)-2,6-dihydropyrrolo[3,4-c]pyrazol-5(4H)-yl]tetrahydro-2H-pyran-3-amine, Omarigliptin
Crystallization from ethyl acetate gave a compound with greater than 99% purity.
Optical rotation [α]D20 −12.0° (c 1.0, CH3OH).
1H NMR (CD3OD, 500 MHz) δ = 1.71 (q, 1H, J = 12 Hz), 2.56–2.61 (m, 1H), 3.11–3.18 (m, 1H), 3.36–3.40 (m, 1H), 3.48 (t, 1H, J = 12 Hz), 3.88–3.94 (m, 4H), 4.30–4.35 (m, 1H), 4.53 (d, 1H, J = 12 Hz), 7.14–7.23 (m, 2H), 7.26–7.30 (m, 1H), 7.88 (s, 1H).
LC–MS: 399.04 (M + 1).
PATENT
Example 1
(2R,3S,5R)-2-(2,5-Difluorophenyl)-5-[2-(methylsulfonyl)-2,6-dihydropyrrolo[3,4-c]pyrazol-5(4H)-yl]tetrahydro-2H-pyran-3-amineStep A: tert-Butyl {(2R,3S,5R)-2-(2,5-difluorophenyl)-5-[2-(methylsulfonyl)-2,6-dihydropyrrolo[3,4-c]pyrazol-5 (4H)-yl]tetrahydro-2H-pyran-3-yl}carbamate
A mixture of Intermediate 2 (26.3 g, 80 mmol) and 2-(methylsulfonyl)-2,4,5,6-tetrahydropyrrolo[3,4-c]pyrazole (Intermediate 5) (15.07 g, 80 mmol) in anhydrous methanol (1.5 L) was stirred at room temperature for 2 h. To the resulting white suspension was added decaborane (2.95 g, 24.15 mmol) and the mixture was stirred at room temperature overnight. Methanol was removed and the residue was purified on two 65i Biotage™ columns eluting with 5-50% ethyl acetate in dichloromethane to afford the title compound as a white solid. LC-MS: 499.10 (M+1).
Step B: (2R,3S,5R)-2-(2,5-Difluorophenyl)-5-[2-(methylsulfonyl)-2,6-dihydropyrrolo[3,4-c]pyrazol-5(4H)-yl]tetrahydro-2H-pyran-3-amine
Removal of the BOC group in the product from Step A (13.78 g, 27.67 mmol) was accomplished with trifluoroacetic acid (100 ml) in dichloromethane (200 mL) at room temperature. After stirring for 2 h, the reaction was concentrated and neutralized with 25% MeOH and 2.5% ammonium hydroxide in dichloromethane. Solvents were removed under reduced pressure and the resulting crude material was purified on a 65i Biotage™ column eluting with 1.25-5% MeOH and 0.125-0.5% ammonium hydroxide in dichloromethane. The isolated material was further purified by recrystallization from 5:1 EtOAc/CH2Cl2 at 60° C. The crystalline product was washed with cold 2:1EtOAc/hexanes to give the title compound as a light brown solid. 1H NMR (500 MHz, CD3OD): 1.71 (q, 1H, J=12 Hz), 2.56-2.61 (m, 1H), 3.11-3.18 (m, 1H), 3.36-3.40 (m, 1H), 3.48 (t, 1H, J=12 Hz), 3.88-3.94 (m, 4H), 4.30-4.35 (m, 1H), 4.53 (d, 1H, J=12 Hz), 7.14-7.23 (m, 2H), 7.26-7.30 (m, 1H), 7.88 (s, 1H). LC-MS: 399.04 (M+1).
Intermediate 2
tert-Butyl[(2R,3S)-5-oxo-2-(2,5-difluorophenyl)tetrahydro-2H-pyran-3-yl]carbamate Step A: 1-(2,5-Difluorophenyl)-2-nitroethanol
To sodium hydroxide (1N, 3L) and methanol (1500 mL) at 5° C. was added a solution of 2,5-difluorobenzaldehyde (350 g, 2.46 mol) and nitromethane (157 mL, 2.9 mol) in methanol (350 mL) dropwise over a period of 1 h. The reaction mixture was then neutralized with glacial acetic acid (165 mL). Diethyl ether (1500 mL) was added and the layers separated. The organic layer was washed successively with saturated aqueous sodium carbonate solution (1000 mL), and saturated aqueous brine (1000 mL). The organic layer was dried over anhydrous magnesium sulfate, filtered and concentrated to afford 1-(2,5-difluorophenyl)-2-nitroethanol that was used without further purification in Step B.
Step B: 2-Nitro-1-(2,5-difluorophenyl)ethanone
A solution of Dess-Martin periodinane (125 g) in dichloromethane (600 mL) was added to a solution of the nitroalcohol made in Step A (46.3 g) at 10° C. over a period of 30 min. Stirring was continued for 2 h, and the reaction mixture was then poured onto a mixture of sodium bicarbonate (300 g) and sodium thiosulfate (333 g) in water (3 L). The desired product was extracted with methyl t-butyl ether (MTBE) (2 L). The aqueous layer was neutralized with HCl (2N, 1.5 L) and extracted with MTBE (3 L). The combined organic layers were dried over anhydrous magnesium sulfate, filtered, evaporated and the residue was purified by chromatography (silica gel, eluting with dichloromethane) to yield the desired nitroketone.
Step C: 3-Iodo-2-(iodomethyl)prop-1-ene
A mixture of 3-chloro-2-(chloromethyl)prop-1-ene (1.0 g, 8 mmol) and sodium iodide (6.6 g, 44 mmol) in acetone (60 mL) was stirred at room temperature for 20 h, evaporated under reduced pressure and partitioned between dichloromethane (150 mL) and water (50 mL). The organic layer was dried over sodium sulfate, filtered and evaporated to yield 3-iodo-2-(iodomethyl)prop-1-ene as a reddish oil.
Step D: 3-Methylene-5-nitro-6-(2,5-difluorophenyl)-3,4-dihydro-2H-pyran
N,N-diisopropylethylamine (184 mL) was added to a solution of 2-nitro-1-(2,5-difluorophenyl)ethanone (92.7 g, 461 mmol) in N,N-dimethylformamide (1000 mL) and 3-iodo-2-(iodomethyl)prop-1-ene (156 g, 507 mmol). The mixture was heated at 60° C. for 2 h, evaporated and purified by chromatography (silica gel, gradient 0-30% dichloromethane in hexane) to yield 3-methylene-5-nitro-6-(2,5-difluorophenyl)-3,4-dihydro-2H-pyran.
Step E: (2R,3S)-5-Methylene-3-nitro-2-(2,5-difluorophenyl)tetrahydro-2H-pyran
This compound was made by following the same method described in Intermediate 1, Step D by using 3-methylene-5-nitro-6-(2,5-trifluorophenyl)-3,4-dihydro-2H-pyran.
Step F: (2R,3S)-5-Methylene-2-(2,5-difluorophenyl)tetrahydro-2H-pyran-3-amine
This compound was made by following the same method described in Intermediate 1, Step E by using (2R,3S)-5-Methylene-3-nitro-2-(2,5-difluorophenyl)tetrahydro-2H-pyran.
Step G: tert-Butyl[(2R,3S)-5-methylene-2-(2,5-difluorophenyl)tetrahydro-2H-pyran-3-yl]carbamate
This compound was made by following the same method described in Intermediate 1, Step F by using (2R,35)-5-methylene-2-(2,5-difluorophenyl)tetrahydro-2H-pyran-3-amine.
Step H: tert-Butyl[(2R,3S)-5-hydroxy-5-(hydroxymethyl)-2-(2,5-difluorophenyl)tetrahydro-2H-pyran-3-yl]carbamate
This compound was made by following the same method described in Intermediate 1, Step G by using tert-butyl[(2R,35)-5-methylene-2-(2,5-difluorophenyl)tetrahydro-2H-pyran-3-yl]carbamate.
Step I: tert-Butyl[(2R,3S)-5-oxo-2-(2,5-difluorophenyl)tetrahydro-2H-pyran-3-yl]carbamate
To a solution of tert-butyl[(2R,3S)-5-hydroxy-5-(hydroxymethyl)-2-(2,5-trifluorophenyl)tetrahydro-2H-pyran-3-yl]carbamate (10.5 g) in methanol (100 mL) at 0° C. was added pyridine (7.8 mL) and lead tetraacetate (21.7 g). The reaction mixture was stirred for 20 min. Aqueous work-up with ethyl acetate gave crude product which was purified by chromatography (silica, 0-50% ethyl acetate/heptane) to yield tert-butyl[(2R,35)-5-oxo-2-(2,5-difluorophenyl)tetrahydro-2H-pyran-3-yl]carbamate as white solid.
Intermediate 3
Step A: tert-Butyl (3Z)-3-[(dimethylamino)methylene]-4-oxopyrrolidine-1-carboxylate
A solution of tert-butyl 3-oxopyrrolidine-1-carboxylate (40 g, 216 mmol) was treated with DMF-DMA (267 g, 2241 mmol) and heated at 105° C. for 40 min. The solution was cooled and evaporated under reduced pressure and the resulting orange solid was treated with hexane (200 mL) and cooled in a refrigerator for 3 days. The resulting brownish-yellow solid obtained as such was collected by filtration, dried and used in the next step without further purification.
Step B: 1,4,5,6-Tetrahydropyrrolo[3,4-c]pyrazole
A solution of hydrazine (3 mL) and tert-butyl (3Z)-3-[(dimethylamino)methylene]-4-oxopyrrolidine-1-carboxylate (19.22 g) in ethanol (40 mL) was heated at 85° C. in a sealed tube for 4 h. Solvent was removed under reduced pressure, and the residue was triturated with dichloromethane (160 mL) and ethyl acetate (15 mL). The resulting solid was filtered. The filtrate was concentrated and the resulting solid was triturated again and filtered. The combined solids were treated with 4N hydrochloric acid (250 mL) in methanol and stirred for 6 h. The reaction mixture was concentrated and dried. The resulting solid was treated again for 6 h with 4N hydrochloric acid (250 mL) in methanol. After concentration and drying, the resulting hydrochloride salt was treated with ammonia in methanol (2N, 300 mL) and ammonium hydroxide solution in water (28%, 30 mL) and concentrated to dryness. The solid obtained was treated with methanol (70 mL) and water (5 mL) and purified in three batches on Biotage Horizon® system (silica, gradient 5-17% methanol containing 10% concentrated ammonium hydroxide in ethyl acetate) to yield 1,4,5,6-tetrahydropyrrolo[3,4-c]pyrazole. 1H NMR (500 MHz, CD3OD): δ 4.04 (d, 4H); 7.39 (s, 1H).
Intermediate 5
2-(Methylsulfonyl)-2,4,5,6-tetrahydropyrrolo[3,4-c]pyrazole Step A: tert-Butyl 1-(methylsulfonyl)]-4,6-dihydropyrrolo[3,4-c]pyrazole-5(1H)-carboxylate (A) and tent-butyl 2-(methylsulfonyl)]-2,6-dihydropyrrolo[3,4-c]pyrazole-5(4H)-carboxylate (B)
A suspension of N-Boc-pyrazolopyrrolidine (Intermediate 3, Step B) (27.16 g, 130 mmol) in anhydrous acetonitrile (1.0 L) was charged in a 2.0 L three-neck flask fitted with a thermometer and an addition funnel and then treated with sodium hydride (60% dispersion in oil, 6.23 g, 156 mmol) while under nitrogen atmosphere in one portion. The reaction mixture was stirred at room temperature for 2 h. The resulting white suspension was then cooled in an ice bath and methanesulfonyl chloride (25.2 mL, 324 mmol) was slowly added via addition funnel The ice bath was then removed and the mixture was stirred 1 h at room temperature. The reaction mixture was quenched with water (500 mL) and the layers were separated. The aqueous layer was then extracted with 2×500 mL of dichloromethane. The combined organic layers were dried over sodium sulfate and concentrated under reduced pressure to give a mixture of products A and B as colorless syrups. NMR in CD3OD indicated a 1:1 mixture of two products, in which the proton on the pyrazole ring in product A appeared at 7.70 ppm while the proton in product B appeared at 7.95 pm. LC-MS: 288.08 (M+1).
Step B: 2-(Methylsulfonyl)-2,4,5,6-tetrahydropyrrolo[3,4-c]pyrazole
Trifluoroacetic acid (200 mL) was added slowly to a solution containing intermediates A and B prepared in the previous step (48.4 g, 168 mmol) in dichloromethane (400 mL) at 0° C. After addition, the cooling bath was removed and the reaction was allowed to stir at room temperature for 2 h. Solvent was removed under reduced pressure and the resulting trifluoroacetate salt was then neutralized with 500 mL of 25% methanol and 2.5% ammonium hydroxide in dichloromethane. After removal of solvent, the desired Intermediate 5 was obtained after chromatography on a Biotage™ column (2×340 g) eluting with 2.5-12.5% methanol and 0.25-1.25% ammonium hydroxide in dichloromethane. LC-MS: 109.85 (M+1).
PATENT
below patent caution................similar not same....examples below will help you in synthesis similarities
Step 1 2 Step 2
Example 1
Synthesis of 3: (Step 1 & 2)
Dimethyldisulfide 1 (5 g, 53 mmol) and acetic acid (6 mL, 106 mmol) were mixed under nitrogen atmosphere and cooled to - 20 °C. Sulfuryl chloride (13 mL, 159 mmol) was added dropwise with stirring. The mixture was then stirred for 1 hour at -20 °C and afterwards allowed to come to room temperature and continued for another two hours. Acetyl chloride was distilled off from the reaction mixture. Crude methanesulfinyl chloride 2 obtained was used in the next step without further purification.
To a solution of chloramine T (14.95 g, 53 mmol) in dry toluene (220 mL) was added a solution of methanesulfinyl chloride 2 (5.2 g, 53 mmol) in dry toluene (10 mL) at 0 °C. The resulting suspension was heated at 80 °C for 2 hours with stirring. After cooling, the solid was filtered off and washed with dry toluene (100 mL). The filtrate was evaporated in vacuo and the crude mixture was purified through silica gel chromatography to obtain 3 as off white solid. XH NMR (300 MHz, CDC13): δ 7.85 - 7.91 (m, J= 8.42 Hz, 2H), 7.31 - 7.38 (m, J= 8.23 Hz, 2H), 3.78 (s, 3H), 2.45 (s, 3H).
Synthesis of 4: (Step 3)
To a solution of Ml (1.0 g, 2.2 mmol) in THF (10 mL) and DMF (10 mL) under nitrogen atmosphere at 0 °C was added Et3N (0.92 mL, 6.6 mmol) followed by B0C2O (0.48 g, 2.2 mmol). The reaction mixture was allowed to come to room temperature and continued the stirring for over night. The reaction mixture was diluted with water (100 mL) and extracted with CH2CI2 (3 x 100 mL). Combined organics were dried over Na2S04, filtered, concentrated under vacuum and purified by silica gel chromatography afforded 4 as a off white solid.
XH NMR (400 MHz, CDC13): δ 7.27 - 7.35 (m, 1H), 4.44 - 4.54 (m, 4H), 1.52 (s, 9H).
Synthesis of 5: (Step 4)
To a suspension of NaH (0.30 g, 7.5 mmol) in dry THF (5 mL) under nitrogen atmosphere at 0 °C was added a solution of 4 (0.78 g, 3.7 mmol) in dry THF (30 mL). The reaction mixture was allowed to come to room temperature and continued the stirring for 2 hours. Reaction mixture was again cooled to 0 °C. A solution of 3 (2.0 g, 7.4 mmol) in THF (25 mL) was added to the reaction mixture and continued the stirring for another 1 hour. The reaction mixture was quenched with water (100 mL) and extracted with EtOAc (3 x 200 mL). Combined organics were dried over Na2S04, filtered, concentrated under vacuum and purified by silica gel chromatography afforded 5 as an off-white solid.
XH NMR (400 MHz, CDC13): δ 7.84 - 7.88 (m, 1H), 7.78 (t, J= 8.27 Hz, 2H), 7.23 - 7.30 (m, 2H), 4.39 - 4.49 (m, 4H), 3.53 (d, J= 2.40 Hz, 3H), 2.42 (s, 3H), 1.53 (s, 9H).; Molecular Formula: Ci8H24N405S2; LCMS purity: 98.18%; Expected: 440.1 ; Observed: 341.0 (M-99).
Synthesis of 6: (Step 5)
To a solution of 5 (0.47 g, 1.06 mmol) in dry CH2CI2 (1 1 mL) under nitrogen atmosphere at 0 °C was added TFA (3 mL). The reaction mixture was allowed to come to room temperature and continued the stirring for 2 hours. Solvent was removed under vacuum and solid mass was washed with Et20 (3 x 10 mL) to get amine TFA salt as white solid.
XH NMR (300 MHz, CD3OD): δ 7.78 (s, 1H), 7.63 - 7.70 (m, J= 8.11 Hz, 2H), 7.26 - 7.35 (m, J = 8.33 Hz, 2H), 3.93 (s, 2H), 3.86 (s, 2H), 3.34 (s, 3H), 2.42 (s, 3H).
The amine TFA salt was dissolved in minimum volume of MeOH:CHCi3 (1 : 1) and passed through a column [Orochem 5 g, 10 ml, Amino (N¾)] using MeOH as eluent.
Organics were concentrated under vacuum to get free 6.
Synthesis of 7: (Step 6) To a stirred solution of 6 (0.34 g, 0.95 mmol) and M2 (0.26 g, 0.79 mmol) in DMAc (6.78 mL) under nitrogen atmosphere for 10 minutes was added AcOH (0.067 mL, 1.19 mmol). The reaction mixture was stirred for further 5 minutes and cooled to 0 °C. NaBH(OAc)3 (0.20 g, 0.95 mmol) was added to the reaction mixture and allowed to stirrer at room temperature for overnight. NH4OH (2 mL) was added to the reaction mixture and heated at 50 °C for 1 hour followed by water (3.39 mL) and again heated at 50 °C for another hour. Reaction mixture was cooled to room temperature and filtered. The solid residue was washed with water (4 x 100 mL) and the crude residue was purified by silica gel chromatography to afford 7.
XH NMR (300 MHz, CDC13): δ 7.80 (d, J= 6.95 Hz, 3H), 7.25 - 7.29 (m, 2H), 7.22 (br. s., 1H), 6.92 - 7.02 (m, 2H), 4.52 (d, J= 9.33 Hz, 1H), 4.24 - 4.40 (m, 2H), 3.85 (br. s., 5H), 3.48 (s, 3H), 3.39 - 3.47 (m, 1H), 3.07 (br. s., 1H), 2.52 (d, J= 10.25 Hz, 1H), 2.44 (s, 3H), 1.61 (br. s., 1H), 1.28 (s, 9H).; Molecular Formula: C29H35F2N506S2; LCMS purity: 99.08%; Expected: 651.2; Observed: 652.0 (M+l). Synthesis of Example 1: (Step 7)
To a solution of 7 (20 mg, 0.03 mmol) in dry CH2CI2 (2 mL) under nitrogen atmosphere at 0 °C was added TFA (0.5 mL). The reaction mixture was allowed to come to room temperature and continued the stirring for 2 hours. Solvent was removed under vacuum and solid mass was washed with Et20 to get amine di-TFA salt Example 1 as white solid. Unless otherwise noted the IC50 values were determined using the assay discussed earlier.
XH NMR (400 MHz, CD3OD): δ 8.05 (s, 1H), 7.73 (d, J= 8.03 Hz, 2H), 7.36 (d, J= 8.28 Hz, 2H), 7.29 - 7.34 (m, 1H), 7.20 - 7.27 (m, 2H), 4.71 (d, J= 10.04 Hz, 1H), 4.40 - 4.53 (m, 5H), 3.72 - 3.82 (m, 2H), 3.68 (s, 3H), 3.59 - 3.65 (m, 1H), 2.77 - 2.85 (m, 1H), 2.44 (s, 3H), 2.00 - 2.14 (m, 1H).; Molecular Formula: C24H27F2 504S2; HPLC purity: 99.74%; LCMS Expected: 551.2; Observed: 552.2 (M+l).
SCHEME 2
Example 2: Synthesis of Compound 1 & 2 (Step 1):
To a suspension of M2 (0.95 g, 2.8 mmol) in water (8.67 mL) was added sodium metabisulfite (0.55 g, 2.8 mmol) and stirred a room temperature for lhour. A solution of M3* (0.52 g, 2.8 mmol) in ethanol (8.67 mL) was added to the above reaction mixture and continued the stirring for further 4 hours. Neat aCN (0.14 g, 2.8 mmol) was added to the above reaction mixture in one portion and heated the reaction mixture at 50 °C for 2 days. Reaction mixture was concentrated under vacuum to remove most of the ethanol. The crude mixture was extracted with CHCI3 (50 x 3 mL). The combined organic layer was washed with water, dried over a2S04, filtered, concentrated and purified by flash chromatography to obtain 1 and 2 as solids.
Compound 1: 'H NMR (300 MHz, CDC13): δ 7.77 (s, 1H), 7.26 - 7.35 (m, 1H), 7.00 (t, J= 5.76 Hz, 2H), 4.57 (t, J= 9.88 Hz, 2H), 4.32 - 4.39 (m, 1H), 3.85 - 4.09 (m, 5H), 3.60 (d, J= 11.34 Hz, 1H), 3.34 (s, 3H), 2.63 - 2.74 (m, 1H), 2.02 - 2.15 (m, 1H), 1.31 (s, 9H).
Compound 2: XH NMR (300 MHz, CDC13): δ 7.28 - 7.36 (m, 2H), 7.00 (t, J= 5.85 Hz, 2H), 4.55 (d, J= 8.97 Hz, 2H), 4.37 (dd, J= 2.65, 11.25 Hz, 1H), 3.88 - 4.07 (m, 5H), 3.60 (d, J = 1 1.34 Hz, 1H), 2.71 (td, J= 3.45, 12.49 Hz, 1H), 1.97 - 2.12 (m, 1H), 1.31 (s, 9H).; Molecular Formula: C22H25F2 503; LCMS purity: 94.48%; Expected: 445.2; Observed: 446.0 (M+l). (*Preparation of M3: M3.PI1SO3H (1.0 g, 2.8 mmol) was dissolved in minimum volume of MeOH:CHCl3 (1 : 1) and passed through a column [Orochem 5 g, 10 ml, Amino (NH2)] using MeOH as eluent. Organics were concentrated under vacuum to get free M3, which was used directly without further purification.) Synthesis of compound 3 (Step 2):
To a solution of compound 2 (0.40 g, 0.89 mmol) in THF (5 mL) under 2 atmosphere at -78 °C was added a solution of MeMgBr (0.89 mL, 2.6 mmol, 3M in Et20). The reaction mixture was allowed to attain room temperature over 1 hour. TLC shows complete conversion. The reaction mixture was again cooled to -10 °C and quenched with saturated aq. NH4CI solution (10 mL). The reaction mixture was extracted with CH2CI2 (50 x 3 mL).
Combined organics were dried over Na2S04, filtered, concentrated and purified by reversed phase chromatography to obtain 3 as di-TFA salt.
Molecular Formula: C22H28F2 4O3; LCMS purity: 88.82%; Expected: 434.2; Observed: 435.2 (M+l).
Synthesis of Example 2 (Step 3):
To a solution of compound 3 (35 mg, 0.053 mmol) in CH2CI2 (2 mL) was added TFA (0.5 mL) dropwise at 0 °C. Reaction mixture was allowed to attain room temperature over 2 hours time. TLC shows complete conversion. Reaction mixture was concentrated to dryness. The solid residue was washed with Et20 (10 x 3 mL) and dried under vacuum to obtain Example 2 as tri-TFA salt.
XH NMR (400 MHz, CD3OD): δ 7.60 (s, 1H), 7.37 (dd, J= 5.02, 8.03 Hz, 1H), 7.22 - 7.31 (m, 2H), 4.70 (d, J= 10.04 Hz, 1H), 4.48 - 4.61 (m, 4H), 4.17 (dd, J= 2.26, 11.29 Hz, 1H), 3.91 (d, J = 11.04 Hz, 1H), 3.73 - 3.83 (m, 1H), 2.54 - 2.62 (m, 1H), 2.22 (t, J= 12.05 Hz, 1H), 1.71 (s, 3H).; Molecular Formula: C17H20F2 4O; HPLC purity: 94.98%; Expected: 334.2; Observed: 335.2 (M+l).
SCHEME 3
Example 3
Synthesis of 1 & 2: (Step 1)
To a suspension of M2 (0.95 g, 2.8 mmol) in water (8.67 mL) was added sodium metabisulfite (0.55 g, 2.8 mmol) and stirred a room temperature for lhour. A solution of M3* (0.52 g, 2.8 mmol) in ethanol (8.67 mL) was added to the above reaction mixture and continued the stirring for further 4 hours. Neat aCN (0.14 g, 2.8 mmol) was added to the above reaction mixture in one portion and heated the reaction mixture at 50 °C for 2 days. Reaction mixture was concentrated under vacuum to remove most of the ethanol. The crude mixture was extracted with CHCI3 (50 x 3 mL). The combined organic layer was washed with water, dried over a2S04, filtered, concentrated and purified by flash chromatography to obtain 1 and 2 as solids.
Compound 1: 'H NMR (300 MHz, CDC13): δ 7.77 (s, 1H), 7.35 - 7.26 (m, 1H), 7.00 (t, J= 5.76 Hz, 2H), 4.57 (t, J= 9.88 Hz, 2H), 4.39 - 4.32 (m, 1H), 4.09 - 3.85 (m, 5H), 3.60 (d, J= 1 1.34 Hz, 1H), 3.34 (s, 3H), 2.74 - 2.63 (m, 1H), 2.15 - 2.02 (m, 1H), 1.31 (s, 9H).
Compound 2: XH NMR (300 MHz, CDC13): δ 7.36 - 7.28 (m, 2H), 7.00 (t, J= 5.85 Hz, 2H), 4.55 (d, J= 8.97 Hz, 2H), 4.37 (dd, J= 2.65, 11.25 Hz, 1H), 4.07 - 3.88 (m, 5H), 3.60 (d, J= 1 1.34 Hz, 1H), 2.71 (td, J= 3.45, 12.49 Hz, 1H), 2.12 - 1.97 (m, 1H), 1.31 (s, 9H).; Molecular Formula: C22H25F2 503; LCMS purity: 94.48%; Expected: 445.2; Observed: 446.0 (M+l).
(*Preparation of M3: M3.PI1SO3H (1.0 g, 2.8 mmol) was dissolved in minimum volume of MeOH:CHCl3 (1 : 1) and passed through a column [Orochem 5 g, 10 ml, Amino (NH2)] using MeOH as eluent. Organics were concentrated under vacuum to get free M3, which was used directly without further purification.) Synthesis of compound 3 (Step 2):
To a solution of 2 (0.40 g, 0.89 mmol) in THF (5 niL) under 2 atmosphere at -78 °C was added a solution of MeMgBr (0.89 mL, 2.6 mmol, 3M in Et20). The reaction mixture was allowed to attain room temperature over 1 hour. TLC shows complete conversion. The reaction mixture was again cooled to -10 °C and quenched with saturated aq. NH4CI solution (10 mL). The reaction mixture was extracted with CH2CI2 (50 x 3 mL). Combined organics were dried over Na2S04, filtered, concentrated and purified by reversed phase chromatography to obtain 3 (0.05 g, 8.4%) as di-TFA salt.
Molecular Formula: C22H28F2 4O3; LCMS purity: 88.82%; Expected: 434.2; Observed: 435.2 (M+l).
Synthesis of compound 4 (Step 3):
To a suspension of NaH (22 mg, 0.55 mmol) in dry THF (0.1 mL) under nitrogen atmosphere at 0 °C was added a solution of 3 (120 mg, 0.27 mmol) in dry THF (4.8 mL). The reaction mixture was allowed to come to room temperature and continued the stirring for 2 hours. Reaction mixture was again cooled to 0 °C. Methanesulfonyl chloride (0.42 mL, 0.55 mmol) was added to the reaction mixture and continued the stirring for another 1 hour. The reaction mixture was quenched with water and extracted with EtOAc (3 x 50 mL). Combined organics were dried over Na2S04, filtered, concentrated under vacuum and purified by silica gel chromatography afforded 4 as off white solid.
Molecular Formula: C23H30F2N4O5S; LCMS purity: 95.64%; Expected: 512.2; Observed: 513.2 (M+l). Synthesis of Example 3: (Step 4)
To a stirred solution of compound 4 (9.0 mg, 0.017 mmol) in CH2CI2 (2.0 mL) was added TFA (0.2 mL) dropwise at 0 °C. Reaction mixture was allowed to attain room temperature over 2 hours time. TLC shows complete conversion. Reaction mixture was concentrated to dryness. The solid residue was washed with Et20 (2 x 10 mL) and dried under vacuum. The solids were once again washed with a mixture of CH2CI2 (0.1 mL) and Et20 (5.0 mL) to obtain Example 3 (8.0 mg, 72.7%) as di-TFA salt. The IC50 value of Example 3 is 4nM. ¾ NMR (400MHz ,CD3OD): δ 7.96 (s, 1 H), 7.41 - 7.31 (m, 1 H), 7.30 - 7.19 (m, 2 H), 4.68 - 4.60 (m, 1 H), 4.22 - 4.07 (m, 4 H), 4.01 (d, J= 11.0 Hz, 1 H), 3.77 (d, J= 11.0 Hz, 1 H), 3.74 - 3.63 (m, 1 H), 3.39 (s, 3 H), 2.43 (d, J= 10.8 Hz, 1 H), 2.04 (t, J= 11.9 Hz, 1 H), 1.51 (s, 3 H).; Molecular Formula: C18H22F2 4O3S; HPLC purity: 95.01%; LCMS mass Expected: 412.2;
Observed: 413.0 (M+l).
PAPER
Org. Process Res. Dev., Article ASAP
DOI: 10.1021/acs.oprd.5b00267
Development of a convergent synthesis of omarigliptin (MK-3102) suitable for commercial manufacture is described. The target molecule is assembled through a diastereoselective reductive amination of a highly functionalized pyranone with a mesylated pyrazole followed by deprotection of a Boc group. The synthesis of the pyranone relies on three Ru-catalyzed reactions: (1) a DKR reduction of a rac-α-aminoketone to set the two contiguous stereogenic centers, (2) a cycloisomerization of a bis-homopropargylic alcohol to a dihydropyran, and, finally, (3) a Ru-catalyzed oxidation of a pyranol to the desired pyranone. The regioselective synthesis of a N-Boc-1-mesyl pyrazole fragment was achieved via base-promoted mesyl group isomerization to afford 30:1 selectivity. A highlight of the endgame process development is telescoping a Boc deprotection and reductive amination followed by direct crystallization of the penultimate from the reaction mixture. This avoids handling of an unstable, mutagenic 1-mesylpyrazole BSA salt used in the earlier multikilogram deliveries and improves the overall diastereoselectivity and efficiency of the route.
Tesfaye Biftu et al, Omarigliptin (MK-3102): A Novel Long-Acting DPP-4 Inhibitor for Once-Weekly Treatment of Type 2 Diabetes;Journal of Medicinal Chemistry, Articles ASAP, March 24, 2014,DOI: 10.1021/jm401992e
Zacuto, Michael J. et al, Process for preparing chiral dipeptidyl peptidase-IV inhibitors;PCT Int. Appl., WO2013003250
Biftu, Tesfaye et al, Novel tetrahydropyran analogs as dipeptidyl peptidase IV inhibitors: Profile of clinical candidate (2R,3S,5R)-2-(2,5-difluorophenyl)-5-[2-(methylsulfonyl)-2,6-dihydropyrrolo[3,4-c]pyrazol-5(4H)-yl]tetrahydro-2H-pyran-3-amine, Bioorganic & Medicinal Chemistry Letters, 23(19), 5361-5366; 2013
Biftu, Tesfaye et al, Preparation of aminotetrahydropyrans as dipeptidyl peptidase IV inhibitors for the treatment or prevention of diabetes,PCT Int. Appl., WO2011028455
Biftu, Tesfaye et al, Preparation of aminotetrahydropyrans as dipeptidyl peptidase IV inhibitors for treatment or prevention of diabetes,U.S. Pat. Appl. Publ., US20100120863
Biftu, Tesfaye et al, Preparation of aminotetrahydropyrans as dipeptidyl peptidase IV inhibitors for treatment or prevention of diabetes,U.S. Pat. Appl. Publ., US20100120863
Xu, Feng et al, Process for preparation of chiral trans-2,3-disubstituted 5-oxotetrahydropyrans from ethyl N-(diphenylmethylene)glycinate and propargyl besylate, U.S. Pat. Appl. Publ., US20090187028
Ru(p-cymene)-N-sulfonyl-l,2-diphenylethylenediamine (DPEN) catalyst
R. Noyori, et al., J. Org. Chem., 66: 7931-7944 (2001)
B. Mohar, et al., Chem. Commun., 2572-2573 (2001)
R. Noyori, et al., J. Org. Chem., 66: 7931-7944 (2001)
B. Mohar, et al., Chem. Commun., 2572-2573 (2001)
The rhodium-catalyzed cycloisomerization
B. Trost etal., J.Amer. Chem.Soc., 125:7482-7483 (2003).
B. Trost etal., J.Amer. Chem.Soc., 125:7482-7483 (2003).
The ruthenium-catalyzed cycloisomerization
B. Trost, et al., J. Amer. Chem. Soc., 124: 2528-2533 (2002)
B. Trost, et al., J. Amer. Chem. Soc., 124: 2528-2533 (2002)
Gantz, I.; Chen, M.; Mirza, A.; Suryawanshi, S.; Davies, M. J.; Goldstein, B. J. Effect of MK-3102, a novel once-weekly DPP-4 inhibitor, over 12 weeks in patients with type 2 diabetes mellitus. Presented at the 48th Annual Meeting of the European Association for the Study of Diabetes (EASD), Berlin, Germany, October 2012; Abstract 101 (Clinical Research, Metabolism, Merck Research Laboratories).

Join me on google plus
Googleplus
amcrasto@gmail.com
Praia dos Galapos
Ns. da Anunciada, 2900 Setúbal, Portugal




Praia de Galápos. Surrounded by lush Mediterranean vegetation Arrábida, the beach is a narrow strip of sand lapped by calm seas, blue and transparent.
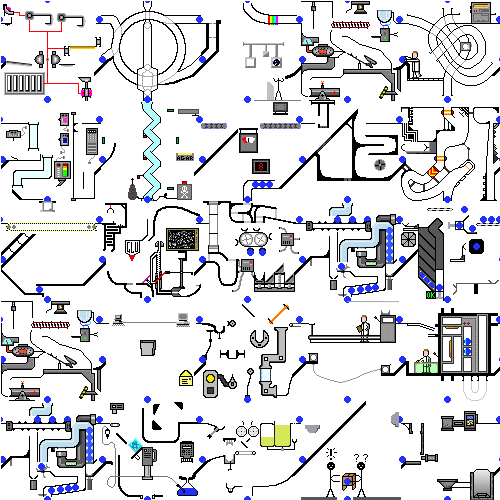
///////
Subscribe to:
Posts (Atom)